![[:en]Bacteria, Symbiosis Mitochondria and their evolutionary role[:]](https://www.gorter-model.org/wp-content/uploads/2016/07/Nature-Education-540x280.jpg)
[:en]Bacteria, Symbiosis Mitochondria and their evolutionary role[:]
[:en]
Robert Gorter, MD, PhD, is emeritus professor of the University of California San Francisco (UCSF)
Bacterias and other micro-organisms play a crucial role in making life possible on earth; No bacterias, no life!
Dr. Robert Gorter: “This article tries to give an overview of the development of bacterias and other micro-organisms and by tracing them back to the earliest stages of evolution and how early bacterias got incorporated into living cells that compose all complex animal organisms up to men. Mitochondria are a perfect example of an early form of a bacteria that was incorporated into a plant or animal cell. Mitochondria, the so-called “powerhouses” of cells, are unusual organelles in that they are surrounded by a double membrane and retain their own small genome. They also divide independently of the cell cycle by simple fission. Mitochondrial division is stimulated by energy demand, so cells with an increased need for energy contain greater numbers of these organelles than cells with lower energy needs (in case of body builders)”
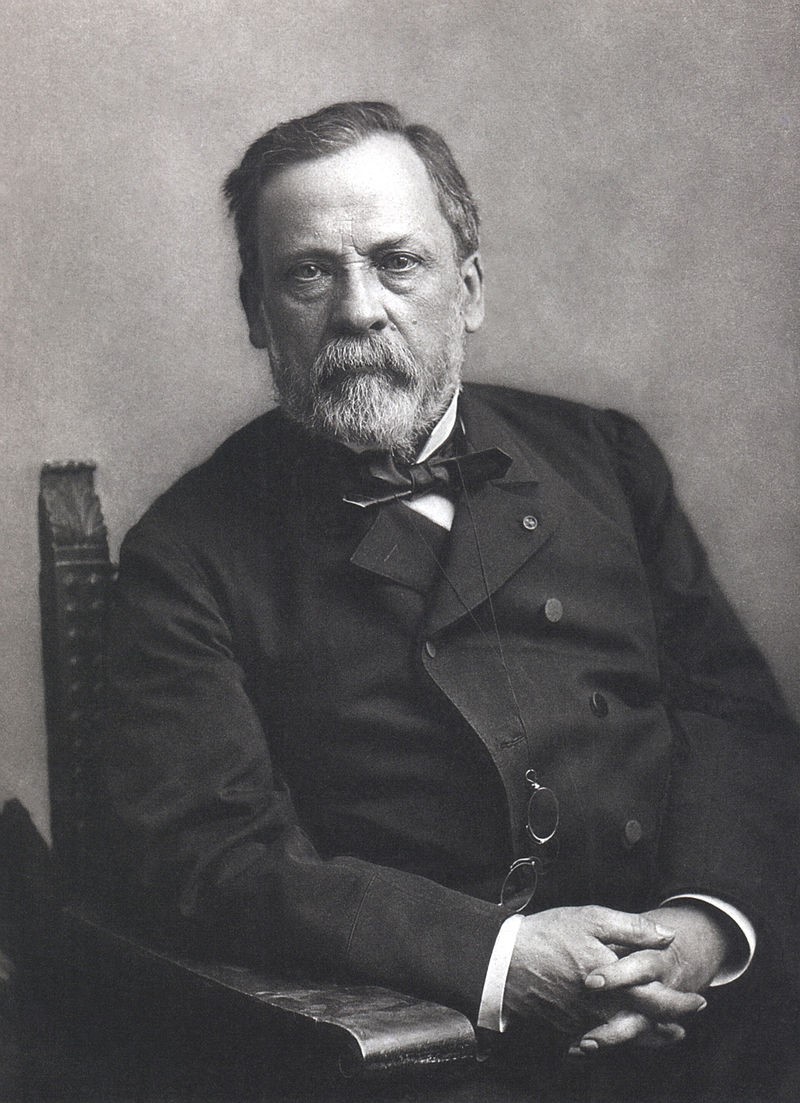
Louis Pasteur (1822-1895) was renowned for his discoveries of the principles of vaccination, microbial fermentation and pasteurization. He is remembered for his remarkable breakthroughs in the causes and preventions of diseases (hygiene)
When Louis Pasteur discovered the pathogens (bacterias and micro-organisms that make us sick) and the principles of sterilization (pasteurization) and hygiene, life expectancy jumped up and quality of life increased significantly.
In modern times, a certain phobia has been developed and has become main stream: an abnormal and persistent fear of bacilli (bacteria). A phobia is an unreasonable and excessive sort of fear that causes avoidance and panic. Phobias are a relatively common type of anxiety disorder in most societies.
Dr. Robert Gorter: “The sterile world people live in industrialized nations pay a prize: increasingly, the incidence and prevalence of severe allergies and autoimmune diseases are documented due to the fact that the immune system is no longer trained by minor infections which are accompanied by fever. Also, the consequent vaccinations against childhood diseases at very early age are associated with decline in overall cellular immune functions, making the adult more vulnerable for malignancies.”
Intracellular Symbioses
Metabolic co-evolution in cooperative symbioses between animals and intracellular bacteria
In this article, Robert Gorter , et. al. wants to give three examples how in evolution various forms of “primitive” live join and compose complex organisms and the biodiversity as we know it. And how Mother Nature can rapidly respond to huge, man-made catastrophes.
Robert Gorter: “About ten to twelve million years ago, a population of African apes diverged down two paths. One lineage gave rise to the gorilla family. The other eventually split again, producing one branch that led to the development of the humans (Homo sapiens) and another that forked into chimpanzees and into bonobos. This is the story of our recent evolutionary past, as far as it is physical.”
It is also the story of some families of microbes living in our guts.
We have tens of trillions of bacteria and other microbes in our guts—at least ten for each of our own human cells that compose the body. Some species within this microbiome are passers-by, which we pick up from our food and our environments. But others are much older companions; and they are our best friends, passed-on to us by breast feeding by our mothers and direct family members.
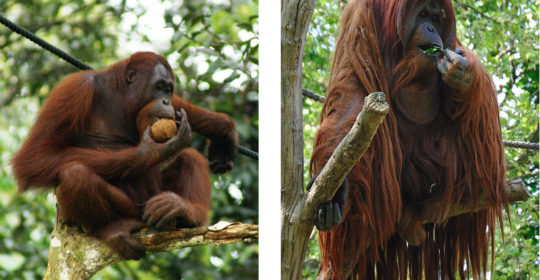
Although orangutans may consume leaves, shoots, and even bird eggs, fruit is the most important part of their diet. They live predominantly in trees
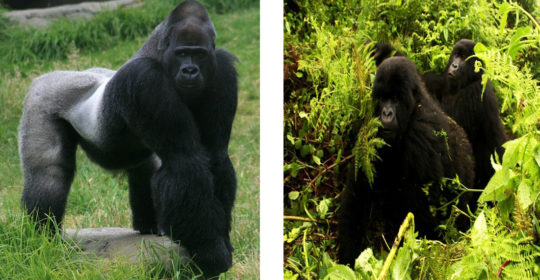
Gorillas are ground-dwelling, predominantly herbivorous apes that inhabit the forests of central Africa
They are the largest living primates. The DNA of gorillas is highly similar to that of humans, from 95–99% depending on what is counted, and they are the next closest living relatives to humans after the chimpanzees and bonobos.
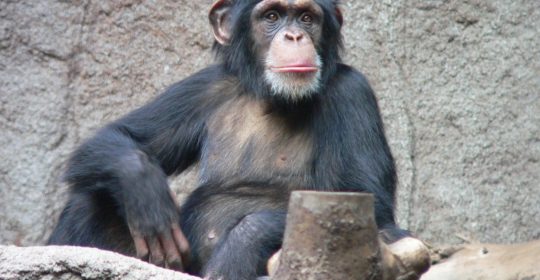
Chimpanzees are one of two exclusively African species of great apes that are currently extant
Native to sub-Saharan Africa, both are currently found in the Congo jungle. Classified in the genus Pan, they were once considered to be one species. However, since 1928, they have been recognized as two distinct species: the common chimpanzee (P. troglodytes) lives north of the Congo River and the bonobo (P. paniscus) who live south. Based on genome sequencing, the two extant Pan species diverged around one million years ago. The most obvious differences are that chimpanzees are somewhat larger, more aggressive and male dominated, while the bonobos are more gracile, very peaceful, and female dominated.
Andrew Moeller, evolutionary biologist at the University of California, Berkeley, USA, found that there are a few groups of human gut bacteria whose history pre-dates humanity. Their ancestors lived in the guts of ancestral apes, and as those ancient animals diverged into modern species, the microbes did, too. In technical terms, they evolved into simpler ones, if you drew out their family tree, you’d get ours for free; you could reconstruct the evolution of apes simply by comparing the right bacteria in their bowels.
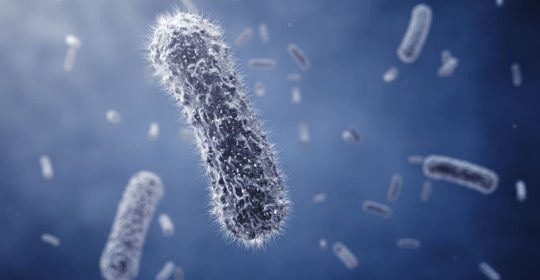
Bacteria are prokaryotes, which consist of a single cell with a simple internal structure
“Some of the bacteria in our gut are derived from very ancient lineages that have been passed down through the primates for millions of years,” says Moeller. “They’re like our genes in that sense.”
Robert Gorter: “Many animals utilize nutritionally-inadequate diets but survive by associating with symbiotic bacteria inside their own cells and that are restricted to specialized insect cells; known as bacteriocytes. Bacteriocytes are invariably transferred from parent to offspring (vertical transmission); and overproduce key nutrients that supplement the inadequate diet of their animal host.”
Currently, investigations are conducted world-wide into how the function of the symbiotic bacteria and the host bacteriocyte are structured for nutrient exchange. We apply metabolic models and experimental approaches, informed by genomic, transcriptomic and proteomic data, to establish how essential amino acid overproduction by the symbiotic bacteria is sustained and scaled to host demand.
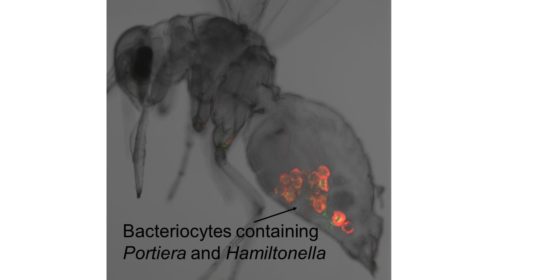
The whitefly Bemisia tabaci, displaying the symbiotic bacteria Portiera (Cy3, red) and Hamiltonella (Cy5, green) in the insect bacteriocytes. FISH micrograph by Hong Wei Shan
Our research concerns both symbioses with a single bacterial partner (e.g. Buchnera in aphids) and symbioses in insects with multiple intracellular symbionts. We are finding that the metabolic capabilities of the bacteriocytes are exquisitely tuned to the nutritional requirements and products of the symbiotic bacteria, including functions that compensate for the loss of metabolism-related genes in the bacteria. In some symbioses, such as the whitefly Bemisia tabaci, the synthesis of certain essential amino acids (e.g. lysine) involves reactions coded by genes horizontally transferred from other bacteria to the insect genome.
Robert Gorter: “Buchnera lacks genes for the biosynthesis of cell-surface components, including lipopolysaccharides and phospholipids, regulator genes and genes involved in defense of the cell. These results indicate that Buchnera is completely symbiotic and viable only in its limited niche, the bacterium. Buchnera is a close relative of Escherichia coli.”
Co-speciation between animals and microbes is fairly common. Aphids, those sap-sucking banes of gardeners, carry Buchnera in them, which provide them with essential nutrients that are missing from their meals. Their alliance was formed between 180 and 250 million years ago, when the dinosaurs were just starting out, and their fates have been entwined ever since. The family trees of aphids and Buchnera strains are also perfect matches.
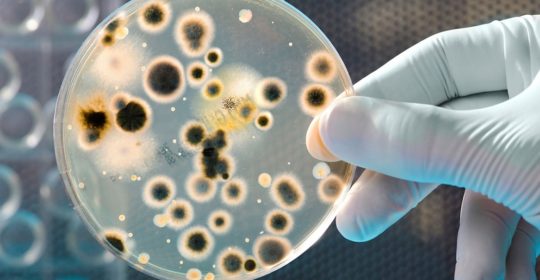
A Petri dish with cultured bacteria’s which usually from colonies when grown in a dish
That’s kind of a special case, though. Buchnera lives inside the cells of its host only, and nowhere else. It would not survive outside a living cell of a host animal. It is also strictly passed from mother to offspring via egg cells. Co-speciation was almost a foregone conclusion. Our gut bacteria have no such constraints. They live freely within the ecosystem of our bowels. They can move vertically from parent to child, but also horizontally from peer to peer. It’s much less obvious that they should have co-speciated with us at all.
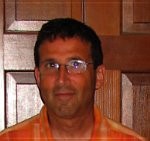
Howard Ochman: Department of Integrative Biology, University of Texas at Austin; Evolutionary Biology, Evolutionary Genetics, Molecular Evolution
In 2010, Howard Ochman found hints that they might. He was the first to show that a family tree constructed using the microbiomes of apes mirrored the one drawn from their own DNA. So, for example, the microbiomes of three chimp subspecies were more similar to each other than to bonobo microbiomes, and more similar to bonobo microbiomes than to human ones.
But Ochman just looked at microbiomes as a whole. Moeller, his graduate student at the time, decided to look for particular species and strains of bacteria whose histories match our own. Moeller relied on a large bank of stool samples, collected from wild apes in Cameroon, Tanzania, and the Democratic Republic of the Congo, and from wild humans living in Connecticut.
Robert Gorter: “Andrew Moeller found many examples of co-speciation within two families of bacteria that are common in our guts—the Bacteroidaceae (“BACK-tuh-roy-DAY-see-ay”) and the Bifidobacteriaceae (“BIH-fih-doh-BACK-tee-ree-AY-see-ay”). Some of the co-speciating microbes, like Bacteroides vulgatus, are familiar to researchers; others are a mystery, and haven’t even been named yet.”
But it’s not as if the entire microbiome is co-speciating neatly, or even most of it. “There are some bacteria that seem to track their host lineage perfectly and others that don’t seem to care which host they’re in—they’re just jumping around,” says Moeller. For example, one lineage had jumped from chimps to gorillas, and another recently moved from humans to chimps.
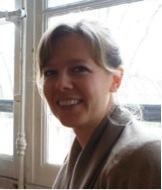
Britt Koskella, University of California, Berkeley, USA, is an evolutionary biologist seeking to understand how interactions and symbioses among species generate and maintain much of the diversity on earth
Still, this study “shows clear potential for the co-evolution between humans and their gut microbiome,” says Britt Koskella, an evolutionary biologist at the University of California, Berkeley, who was not involved in the study. “It also raises interesting questions about how modern human behavior, such as cleanliness and changes in diet, might be altering those tight associations and leading to increased health problems, like allergies and cancer.”
Robert Gorter: “Koskella is talking about the hypothesis, popularized by Martin Blaser, that we are inadvertently evicting longstanding bacterial partners from our bodies. Moeller certainly found some evidence for this. He showed that some lineages of co-speciating bacteria are present in gorillas, chimps, and bonobos, but their counterparts are missing from Western guts (although some still exist within people living in rural Malawi).”
That raises two important questions:
Firstly, “why are these microbes disappearing?” Dr. Robert Gorter and others have blamed the trappings of modern life, including wanton use of antibiotics and an obsession with sanitation. That’s possible, but in a previous study, Andrew Moeller showed that the diversity of the human gut microbiome has been falling for a very long time; it’s lower among human hunter-gatherers than chimps or gorillas, and then still lower still among Western city-dwellers. “I think it probably started when humans started cooking our food,” he says. “Immediately, you’re going to lose some ability to host a complex consortium of bacteria, because you don’t need them anymore.”
Secondly, “Are these lost groups doing important things in chimps and bonobos, which we’re not getting?” Moeller asks. Some would argue that these microbes must have been doing something important, given their long history with us. Robert Gorter: “likely, they helped to digest our food or calibrate our immune system, and perhaps their absence partly explains why chronic inflammatory and autoimmune diseases (and also cancer?) are on the rise.
It’s a compelling idea, but a speculative one. We still don’t really know how these ancient microbes are affecting us, or vice versa. Which means, according to Katherine Amato, from Northwestern University, that we can’t strictly claim that we’re co-evolving with them. “Do these patterns of co-speciation matter to us in the grand scheme of natural selection?” she asks. “Does having the wrong strain make individuals less reproductively successful?” Jessica Metcalf, from the University of Colorado in Boulder, has similar questions. “What do bacteria that co-diversified with their hosts have in common?” she asks. “And are they more important to their hosts than ones that were acquired through the environment?”
“When we start to be able to answer these questions is when things will really get exciting,” says Amato. To do that, she thinks researchers will need to look at the entire genomes, to see if changes in the microbes correlate with new traits or abilities in their hosts. They’ll also need to move outside the apes and think about monkeys, lemurs, and other primates. “Searching for patterns among only four host species may be over-simplifying aspects of the story,” she says.
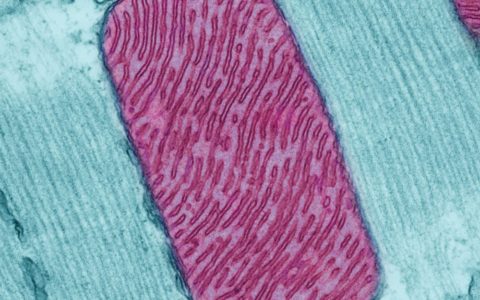
Mitochondria (red) from heart muscle cell of a rat. Nearly all our cells have these structures. Credit: Thomas Deerinck, National Center for Microscopy and Imaging Research
Mitochondria are specialized structures unique to the cells of animals, plants and fungi. They serve as batteries, powering various functions of the cell and the organism as a whole. Though mitochondria are an integral part of the cell, very strong evidence shows that they evolved from primitive bacteria which were incorporated very early on in cells of primitive life.
All living organisms are built with one fundamental brick: the cell. In some cases, a single cell constitutes an entire organism. Cells contain genetic material (DNA and RNA), and they carry out essential functions, such as metabolism and protein synthesis. Cells are also capable of self-replicating. However, the level of organization varies within the cells of different organisms. Based on these differences, organisms are divided into two groups: eukaryotes and prokaryotes.
Plants, animals and fungi are all eukaryotes and have highly ordered cells. Their genetic material is packaged into a central nucleus. They also have specialized cellular components called organelles, each of which executes a specific task. Organelles such as the mitochondria, the rough endoplasmic reticulum and the golgi complex serve respectively to generate energy, synthesize proteins and package proteins for transport to different parts of the cell and beyond. The nucleus, as well as most eukaryotic organelles, is bound by membranes that regulate the entry and exit of proteins, enzymes and other cellular material to and from the organelle.
Individual mitochondria are capsule shaped, with an outer membrane and an undulating inner membrane, which resembles protruding fingers. These membranous pleats are called cristae, and serve to increase the overall surface area of the membrane. When compared to cristae, the outer membrane is more porous and is less selective about which materials it lets in. The matrix is the central portion of the organelle and is surrounded by cristae. It contains enzymes and DNA. Mitochondria are unlike most organelles (with an exception of plant chloroplasts) in that they have their own set of DNA and genes that encode proteins.
Prokaryotes, on the other hand, are single-celled organisms such as bacteria and archaea. Prokaryotic cells are less structured than eukaryotic cells. They have no nucleus; instead their genetic material is free-floating within the cell. They also lack the many membrane-bound organelles found in eukaryotic cells. Thus, prokaryotes have no mitochondria.
Mitochondria Function
The main function of mitochondria is to metabolize or break down carbohydrates and fatty acids in order to generate energy. Eukaryotic cells use energy in the form of a chemical molecule called ATP (adenosine triphosphate).
ATP generation occurs within the mitochondrial matrix, though the initial steps of carbohydrate (glucose) metabolism occur outside the organelle. According to Geoffrey Cooper in “The Cell: A Molecular Approach 2nd Ed” (Sinauer Associates, 2000), glucose is first converted into pyruvate and then transported into the matrix. Fatty acids on the other hand, enter the mitochondria as is.
ATP is produced through the course of three linked steps. First, using enzymes present in the matrix, pyruvate and fatty acids are converted into a molecule known as acetyl-CoA. This then becomes the starting material for a second chemical reaction known as the citric acid cycle or Krebs Cycle. This step produces plenty of carbon dioxide and two additional molecules, NADH and FADH2, which are rich in electrons. The two molecules move to the inner mitochondrial membrane and begin the third step: oxidative phosphorylation. In this last chemical reaction, NADH and FADH2 donate their electrons to oxygen, which leads to conditions suitable for the formation of ATP.
Dr. Robert Gorter: “Logically, mitochondria multiply when a the energy needs of a cell increase. Therefore, power-hungry cells have more mitochondria than cells with lower energy needs. For example, repeatedly stimulating a muscle cell will spur the production of more mitochondria in that cell, to keep up with energy demand. Logically, mitochondria multiply when the energy needs of a cell increase. Therefore, power-hungry cells have more mitochondria than cells with lower energy needs. For example, repeatedly stimulating a muscle cell will spur the production of more mitochondria in that cell, to keep up with energy demand.”
A secondary function of mitochondria is to synthesize proteins for their own use. They work independently, and execute the transcription of DNA to RNA, and translation of RNA to amino acids (the building blocks of protein), without using any components of the cell. However, here too, there are differences within eukaryotes. The sequence of three DNA nucleotides U-A-G (uracil-adenine-guanine) is an instruction for translation to stop in the eukaryotic nucleus.
Origins of mitochondria: The Endosymbiont Theory
In her 1967 paper, “On the Origins of Mitosing Cells,” published in the Journal of Theoretical Biology, scientist Lynn Margulis proposed a theory to explain how eukaryotic cells along with their organelles were formed. She suggested that mitochondria and plant chloroplasts were once free-living prokaryotic cells that were swallowed up by a primitive eukaryotic host cell.
Lynn Margulis’ hypothesis is now known as the “endosymbiont theory.” Dennis Searcy, emeritus professor at University of Massachusetts Amherst, explained it as follows: “Two cells began to live together, exchanging some sort of substrate or metabolite (product of metabolism, like ATP). The association became mandatory, so that now, the host cell cannot live separately.”
Even at the time that Margulis proposed it, versions of the endosymbiont theory were already in existence, some dating back to 1910 and 1915. “Although these ideas are not new, in this paper they have been synthesized in such a way as to be consistent with recent data on the biochemistry and cytology of subcellular organelles,” she wrote in her paper. According to a 2012 article on mitochondrial evolution by Michael Gray in the journal Cold Spring Harbor Perspectives in Biology, Margulis based her hypothesis on two key pieces of evidence. First, mitochondria have their own DNA. Second, the organelles are capable of translating the messages encoded in their genes to proteins, without using any of the resources of the eukaryotic cell.
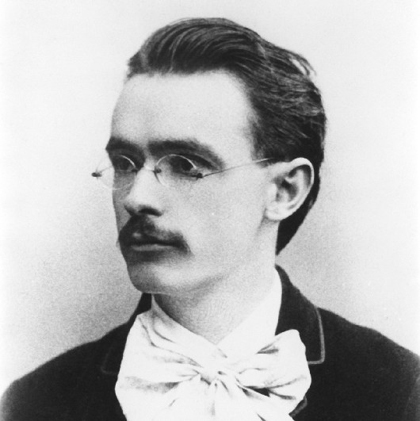
Rudolf Steiner (1861-1925)
Already in Berlin in 1906 and during the following years, the German scientist Rudolf Steiner pointed out that earlier, more primitive life forms merged anatomically as well as functionally (physiologically) and building the anatomy of new, higher-developed organisms; guided by evolutionary dynamics (but not ad random) which then developed into the biodiversity as we know today.
Genome sequencing and analyses of mitochondrial DNA have established that Margulis was correct about the origins of mitochondria. The lineage of the organelle has been traced back to a primitive bacterial ancestor known as alphaproteobacteria (α-proteobacteria).
Robert Gorter: “Despite the confirmation of the mitochondria’s bacterial heritage, the endosymbiont theory continues to be researched. One of the biggest questions stays, ‘Who is the host cell?’”
The questions that linger are whether mitochondria originated after the eukaryotic cell arose (as hypothesized in the endosymbiont theory) or whether mitochondria and host cell emerged together, at the same time (this theory is strongly supported by Rudolf Steiner).
Bacteria: The First Responders to Oil Spills
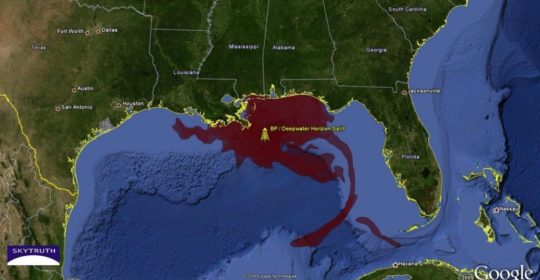
This map shows the magnitude of the oil spill in the Gulf of Mexico
The toxic substance, composed of alkanes and aromatic hydrocarbons, posed a huge threat to the organisms that called the Gulf their home. Not only did the oil congeal on the surface, but it also settled into the ocean’s sediment. It spread literally everywhere.
In a desperate attempt to reverse the damage, dispersants were distributed alongside the oil. At that point in time, the scientific community and the cleanup crew were unaware that certain bacterial species had already appeared at the scene instead of fleeing it.
That’s right – among the estimated 1 trillion microbial species that inhabit the globe, several species of bacteria actually use their natural metabolic processes for good purposes. They can reduce the amount of oil waste spilled during disasters such as the Deepwater Horizon Spill.
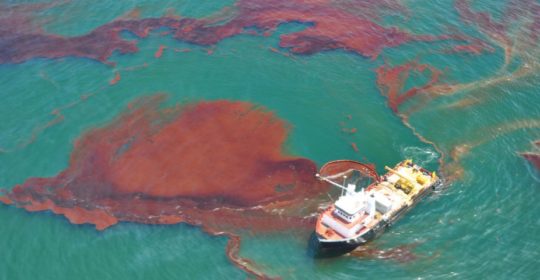
In 2010, in an event now known as the Deepwater Horizon Spill, the Gulf of Mexico’s fragile oceanic ecosystem became severely polluted with approximately 4.9 million barrels of oil
In a recent publication written by a collaboration of researchers from The University of Texas Marine Science Institute, the University of North Carolina, and Heriot-Watt University, it was suggested that these bacteria have special genes which allow them to quickly adapt to environments with low nutrients.
Their gene sequencing, published in the journal Nature Microbiology, was done in order to further understand the relationship between toxic spills and bacterial function.
One bacteria species in particular, Neptuniibacter, had never before surfaced at an oil spill scene. Yet there it was – working closely alongside the more commonly present Alcanivorax to feast heartily on the oil.
Interestingly, the researchers found that over time the sudden rush of chemicals changed the complete structure of the microbial community in the area. When they returned to the spill site after one year, they noted that the bacterial communities had become more diverse over the time period.
The conclusions drawn from the research show just how adaptable the bacteria are. Their ability to modify themselves to fit a suddenly altered environment is a remarkable characteristic.
Unfortunately, oil spills are common in the Gulf, and elsewhere. But, luckily, bacterias native to the ecosystem are prepared and ready for action. Think of these small yet mighty organisms as first-responders to the scene of the accident. They assess the damage and do what they can to stabilize the area. Then, they call for backup.
“After the spill, all bacteria work(ed) together to efficiently degrade oil,” commented Nina Dombrowski, a post-doctoral researcher who contributed to the research.” Bacterial communities already present at the site of an oil spill respond(ed) in a rapid and efficient manner, becoming abundant during the spill and actively degrading oil compounds.”
As we get to know the significance of these microorganisms, we can promote understanding and conservation of the microbial world. This knowledge also provides us with helpful insight to reversing the damage done to marine ecosystems.
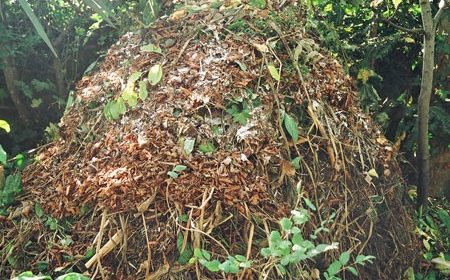
Compost heap in a garden
Composting: its importance and the role of bacteria and other micro-organism
Robert Gorter: “Bacteria are found in every living habitat on earth and play an essential role with regards to composting. In fact, without compost bacteria, there would be no compost, or life on planet earth for that matter. Beneficial bacteria found in garden compost are the “garbage men” of the earth, cleaning up trash and creating a useful product. In other words, they recycle almost anything that is bio degradable.”
Bacteria are able to survive extreme conditions where other life forms crumble. In nature, compost exists in areas such as the forest, where compost-enhancing bacteria decompose organic matter like tree and animal droppings but also carcasses. Putting beneficial bacteria to work in the home garden as well as in agriculture is an environmentally friendly practice that is well worth the effort.
Beneficial bacteria found in garden compost are busy breaking down matter and creating carbon dioxide and heat. The temperature of compost can get up to 60 degrees Celsius due to these heat-loving microorganisms. Compost-enhancing bacteria work around the clock and in all sorts of conditions to break down organic material. In tempered climates, during frost the composting processes are slowed down but not come to a complete stop.
Fungi
Fungi plays important role as decomposing of complex plant polymers in soil and compost. Fungi include molds and yeasts, in compost they break down tough debris, enabling bacteria to continue the decomposition process once most of the cellulose has been exhausted. Fungi grows rapidly by producing many cells and filaments, and they can attack organic residues that are too dry, acidic, or low in nitrogen for bacterial decomposition. Saprophytes because they live on dead or dying material and obtain energy by breaking down organic matter in dead plants and animals. Fungi are mostly found during both mesophilic and thermophilic phases of composting. During higher temperature they live on outer layer of compost. Scytalidium thermophilum helps in growth of thermophilic fungus.10 Fungi favor an acidic pH range.
Actinomycetes
Actinomycetes are fungi resembled bacteria with filamentous. They are resistant to dry, acidic, or low in nitrogen for bacterial decomposition environment. Saprophytes because they live on dead or dying material and obtain energy by breaking down organic matter in dead plants and animals. Variety of Fungal Species are found during both mesophilic and thermophilic phases of composting. During higher temperature they like to live in the outer layer of compost.
Protozoa
Protozoa are one-celled microscopic animals. Mostly found in water droplets in compost but play a relatively minor role in decomposition. Protozoa obtain their food from organic matter in the same way as bacteria do but also act as secondary consumers ingesting bacteria and fungi.
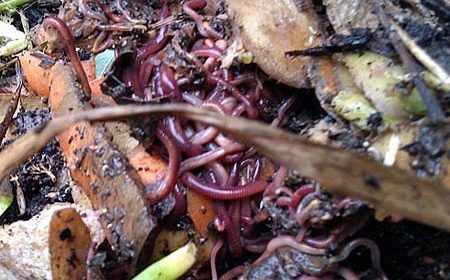
Worms and other invertebrates are very essential in the overall composting or organic materials
Other Invertebrates
Ants make compost richer in phosphorus and potassium by moving minerals from one place to another. Springtails one of the most important invertebrates as they help in breaking down materials They chew on decomposing plants, pollen, grains, and fungi. They also like to eat nematodes and droppings of other arthropods and then clean themselves after feeding. Snails and slugs like fresh garbage so mostly found on top of compost pile. Centipedes are fast moving predators found mostly in the top few inches of the compost heap. They have formidable claws behind their head which possess poison glands that paralyze small red worms, insect larvae, newly hatched earthworms, and arthropods (mainly insects and spiders). Files are mostly found in mesophilic stage, they provide airborne transportation for bacteria. They also eat organic vegetation. Files larval phase (maggots) does not survive thermophilic temperatures.
Their incorporation into soil increases fertility (e.g. nitrogen fixers, nitrifiers, sulphur oxidizers), structure (e.g. exopolysaccharide producers), and can have other effects as a result of high activity and population levels, as well as through specific biochemical traits of the micro-organisms. Increased numbers of microorganisms in compost also have an indirect role in improving plant health. Microorganisms form symbiotic associations with plant roots and synthesize and excrete nutrients (amino acids, vitamins), plant growth hormones and chelators, alter physical conditions to optimize plant growth, and decompose or neutralize toxic substances. The majority of bacteria isolated from composts were Gram-negative.7 Microorganisms may affect negatively as they compete for oxygen, nutrient which can inhibit metabolic products. Optimal pH values for composting range from pH 5.5 to 8.0. Initial pH of composting are acidic which forms acid-forming bacteria. As pH starts to increase and becomes alkaline and finally drops back to near neutral as a result of humus formation.
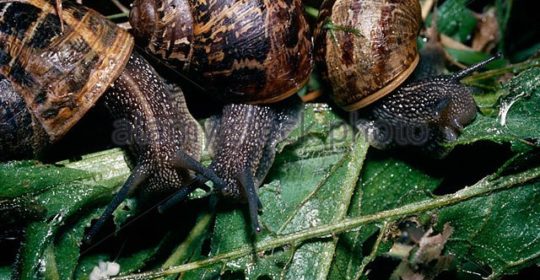
Garden snails (Helix aspersa: Helicidae) on a compost heap feeding
Once decomposed, this rich, organic dirt is used in the garden and in agriculture to enhance existing soil conditions and improve the overall health of plants that are grown there.
References:
Extension.oregonstate.edu/…/MicroorgansimsInvolvedincomposting.pdf
www.genomenewsnetwork.org/articles/09_00/Buchnera.shtml
https://www.youtube.com/watch?v=pcXdfofLoj0
www.nature.com/articles/35024074
[:]